Sleepy puppies, fish and flies
- ScienceDuuude
- Jun 19, 2020
- 12 min read
Updated: Jun 21, 2020
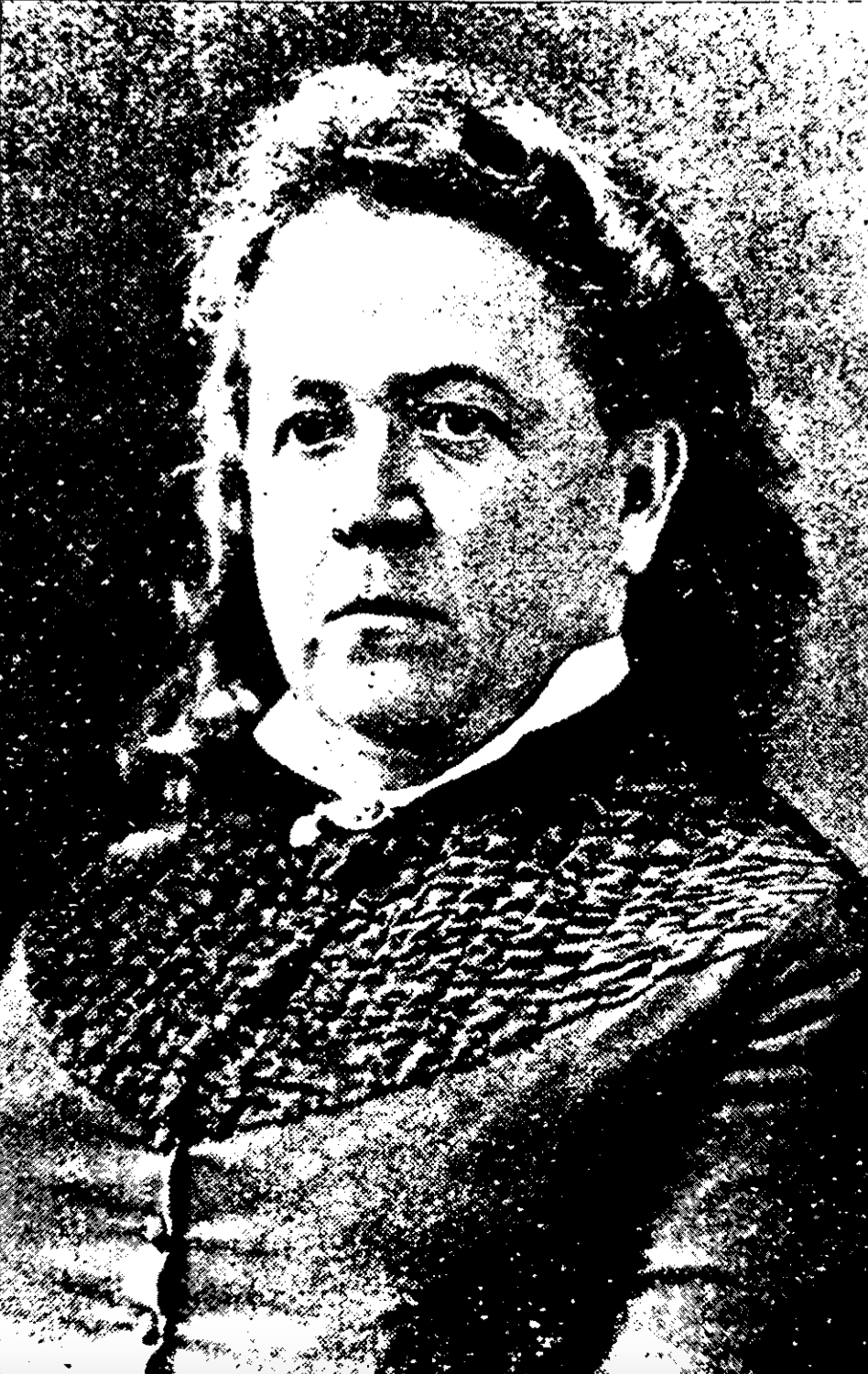
Sometime in the early 1870s in Saint-Petersburg a Russian physician-scientist named Maria Mikhailovna Manasseina was conducting research on the essential nature of sleep using a basket of 2- to 4-month-old puppies. The sentimental holiday card image of this idyllic project involving a pile of puppies soon turns ominous as we see that Manasseina and her assistants worked around the clock to keep the puppies relentlessly active and awake. If the horror escapes you, recall that sleep deprivation is a universal method of torture from ancient China to modern police states and black ops all across the world. As the enforced sleep deprivation continued day after day, the puppies’ temperatures began to drop, their blood began to thicken, their reflexes slowed, and they became weak and floppy. The puppy’s pupils eventually became non-responsive or slow to react to light, and despite feeding more and more from their mothers, they lost weight. After four to five days of total sleep deprivation, the puppies died. Manasseina noted that sleep was more essential than food, as puppies could withstand 20-25 days without food (another experiment she conducted) and still be restored to health with gentle restoration of feeding. But puppies totally deprived of sleep for 4-5 days could not be rescued. They all died.
This horrendous study was our first direct experimental proof that sleep is an essential biological function, yet to this day we still do not understand how or why that is.
If we are lucky, we spend a third of our lives asleep. Eight hours out of a day. Thirty-three years out of a century. For something that we spend so much time on, we hope it is important, useful, even essential. Many work-places today require multiple or extended shifts and jigger with workers' sleep-schedules, so it is important to understand this basic biological process and how we might manage the ill effects of disrupting it. The fact that many auto accidents and fatalities may be caused by sleep deprivation suggests that managers may be playing with fire, with their employee’s lives, when they casually juggle shift schedules.
Sleep is biologically important, if we use the variety of animals that sleep as one measure for how fundamentally important it is. The question we might ask is: how conserved is sleep across the range of animal species. We believe mammals and birds sleep, for example, because, well… we see them sleeping. Everything from bats hanging upside down, their wings wrapped about themselves to form leathery cocoons, to sperm whales sleeping vertically, like huge blubbersome columns holding up an Atlantean temple, to birds on a wire, head tucked under a wing as if whispering secrets into their feathers… We see all these animals close their eyes and settle down, we see them fall into a stupor, their breathing slows down, muscles slacken, they become relatively unresponsive to all but extreme stimuli, sometimes their limbs or bodies twitch or make running movements while prone - and we’ll point to our puppy and say that they are dreaming. We catalog what we believe are behavioral markers for sleep in ourselves and apply them to other species – and we recognize animal sleep behaviors as similar to our own.
Another common and perhaps more analytical method of determining if something is asleep is to record brain and/or muscle activity. Tools such as electroencephalography (EEG) and electromyography (EMG) record patterns of brain or muscle activity that we can then identify as typical of sleep or wakefulness. Sleep researchers have further sub-divided mammalian sleep into two states discernable by EEG: rapid eye movement (REM) sleep, and non-REM (NREM) sleep. One study by researchers from Université Claude Bernard in Lyon, France used EEG to extend the groups of animals with identifiable mammalian-like two-state sleep patterns to lizards, and suggested that “these two [sleep] states arose with the common ancestor of mammals, birds, and reptiles”.
In addition to common behavioral and electrical characteristics that we use to define sleep in larger organisms, some surprising molecular mechanisms also appear to have been evolutionarily conserved across species. Molecules such as salt-induced kinases (SIK3), epidermal growth factor, cyclic adenosine monophosphate, melanin, melatonin and others have been implicated in sleep’s molecular pathways that are conserved across an incredibly broad range of species. Animals that look nothing like us share with us a complex behavior like sleep along with the molecular pathways that control sleep (which then opens the door to ask what other complex behaviors do we share with all animals).
Although it is possible to obtain EEG or EMG recordings from smaller and simpler animals than mammals and birds, it becomes technically challenging and expensive, so other methods become prominent. Combinations of electrical with behavioral and molecular studies become necessary with animals like one of biology’s favorite model organisms, the zebrafish. One such study at Stanford University found that the two-state sleep behavior extended to fish and they concluded that “neural signatures of sleep may have emerged in the vertebrate brain over 450 million years ago”. The fascinating thing about this study is how they genetically modified zebrafish to express fluorescent signals in place of using electrodes as a way to record brain electrical activity – and in this way were able to use non-invasive microscopy to show that these fish’s brains display a two-state sleep similar to mammals and birds. Furthermore, the Stanford researchers showed that like mammals, zebrafish also use melanin and melanin concentrating hormone as key regulators of sleep.
The brain has been the obvious focus of sleep research, and the assumption has been that sleep is a characteristic behavior in creatures with a central nervous system or brain. So we watch with fascination as scientists discover more and more animals with smaller and simpler central nervous systems that continue to display sleep-like behaviors and characteristics.
A combination of behavioral and molecular methods is necessary to detect sleep in even simpler organisms such as fruit flies (Drosophila melanogaster) and round worms (Caenorabditis elegans), two other very commonly used model laboratory organisms. Studies using these combination methods have shown that even simple organisms like fruit flies and round worms exhibit behaviors that indeed look very much like sleep. Fly researchers found that several molecular markers of sleep behavior in mammals also operate in flies. The worm researchers, in turn, found that genes implicated in sleep-like behaviors in flies also operated in worms, and suggested that “this sleep-like state has a role in growth and development…promotes nervous system change.”
In 2017, a study at the California Institute of Technology showed that jellyfish, which do not have brains, also showed sleep-like behavior, suggesting, as the authors say, that “sleep arose early in the metazoan lineage, prior to the emergence of a centralized nervous system”. The authors note that melatonin is a highly conserved molecule that has been shown to promote sleep in vertebrates from zebrafish to humans, as well as in invertebrates, and their data showed that melatonin also induced sleep-like behavior in Cassiopea, the jellyfish they studied.
So, sleep is important and arose very early in evolution. Before mammals, birds, lizards, or amphibians, before vertebrates, before jawed-fish, before a bilateral (left-right mirror-imaged) body plan, and even before brains… sleep (and the molecular pathways that induce sleep) appears to be so important that it has been with us since the dawn of complex multicellular animals.
Although it is fascinating to know through these studies that sleep is a common behavior in everything from jellyfish to humans, we still don’t know how sleep is important to such a wide array of species, and why it has been conserved through all this evolutionary time. We infer sleep’s importance from the fact that it has been so well conserved.
(By the way, the value of science goes far beyond the gee-wizzery and fascination of knowing that a jellyfish shares molecular pathways and behaviors with human sleep. Although knowledge does have tremendous value for its own sake, it is much more important than that. The knowledge of highly conserved molecular pathways in sleep, for example, gives us the ability to experimentally test drugs and therapies in animals much more quickly and thoroughly before they come to human trials. But for animal studies to have any chance of being acceptable proxies for humans, we need to understand their genetic and molecular details and understand where they are similar and where they differ. This of course leaves unsaid the ethical quandary of using animals in experiments that we would never perform on humans.)
One way to determine how important something is and whether it is essential, is to remove it and see what happens. This was the basis for Maria Manasseina’s sleep deprivation study with puppies. What Manasseina found was that sleep is biologically essential because if we deprive puppies of sleep, they die in less than a week. But is this also true for humans?
It turns out that there is a human condition called Fatal Familial Insomnia (FFI) that sheds some light on the essential nature of sleep. FFI is an incurable inherited disease caused by a mutation in the gene called Prion Protein (PrP). People with FFI suffer worse and worse insomnia and eventual death, usually within months after the onset of symptoms.
There is a fascinating two-part case study (published here and here) of a 52-year-old white American male with a doctorate in naturopathy. This energetic and enthusiastic patient, known only as DF, attempted to self-manage his FFI symptoms and appeared to extend his survival time by almost a year through various methods, most prominently a rotation of sleep medications and stimulants about 15 months into his illness, but also with vitamin supplements and exercise early in the course of the disease. He wrote a book and drove a motorhome across the US and befriended FFI researchers with his charm and humor. But eventually DF, like all others with this disease, died.
But does FFI really prove that lack of sleep causes death – and that sleep is essential? Isn’t it possible that the mutation in PrP could gum up the works in other essential biological functions, and that insomnia happens to be a side-effect of the disease? Unfortunately, autopsies of patients with FFI tend to focus on the brain, since accumulation of prions and lesions in the brain is the classic marker of related prion diseases such as mad cow disease and Creutzfeld-Jakob Disease. For now, we don’t know, and FFI remains a rare and fatal genetic disease which hints at the essential nature of sleep.
Over the last hundred years after Manasseina’s morbidly inspiring experiments, many scientists have tried to understand the biological role of sleep by depriving laboratory animals of sleep and analyzing the effects. Manasseina conducted autopsies on the puppies and found no obvious signs of degradation in any organ system except the brain where she noted some pathological changes. The one thing we have learned over the past century is that all animals tested to date, from invertebrates like flies and worms on up to humans (if we include the informal experiments conducted in torture chambers around the world), all die when deprived of sleep.
The ranks of researchers following Manasseina have performed variations on her studies into the early decades of the 20th century, using various methods of keeping animals constantly awake until they perished, and using various methods to analyze the organs and cells of the deceased animals. Their results were fundamentally no different from hers. Older dogs, for example, were more resilient than puppies but they eventually succumbed as well, and the anatomic cause of death remained elusive. Aside from the inconclusive nature of the results, a key weakness in all these studies was the inability to clearly point to the cause of death – was it lack of sleep or was it because of excessive stress? Was the stress, caused by the researcher constantly prodding the experimental subject to remain awake, the actual cause of death rather than lack of sleep?
To address this lack of experimental control, Allan Rechtschaffen’s lab at the University of Chicago performed a then-novel sleep deprivation study using rats in 1989. A turntable centered between two cages kept a rat in each cage out of water, and remained stationary when the rats were active. But the turntable automatically rotated and forced both rats into the water only if the experimental rat started to fall asleep, as measured by an electrode implanted in its brain. The control rat could sleep whenever the experimental rat was awake. All ten experimental rats in the study (aged 4-6 months) died within 11-32 days (normal average life span is about 3 years). The control rats remained healthy and survived. Autopsies showed that there were no obvious anatomic causes of death, and since both experimental and control rats were subjected to the same levels of immersion and physical activity or stress, the researchers could link the amount of sleep to the difference in lethality. This experiment seemed to give us the best answer to our question to date: lack of sleep does indeed cause death.
But how?
Rechtschaffen’s rats, just as with Manasseina’s puppies, displayed few clear anatomical signs of damage from lethal sleep deprivation, and researchers continued to focus on the brain above all else for clues.
Many studies ask what normal biological processes are affected by less extreme sleep deprivation (or conversely what genes or biological processes, when altered, disrupt normal sleep). Using such methods, sleep has been associated in recent studies with energy conservation, temperature regulation, toxin removal from neurons, tissue restoration, and learning and memory among other biological processes. And sleep may well have evolved multiple important biological roles as life emerged from the muck of a stagnant pond and stretched toward a prehistoric sun or sprinted savagely toward its herd of thundering prey across the fern-studded landscape. But understanding which, if any of these processes are linked to the original or essential nature of sleep has continued to stymie the sleep field.
One of the more interesting linkages is between sleep, microbial infections, and the gut. A fascinating study at the University of Tennessee examined the effects of staphylococcus aureus infections on sleep in rabbits, and found that changes in sleep (increased NREM and decreased REM sleep) may be an adaptive response to infections. Going the other direction, sleep deprivation was shown to impair host immune defenses leading to increased bacterial invasion of the host through the gut lining.
A new study done at the Harvard Medical School suggests a compelling link between sleep, the gut, and the lethality of extreme sleep deprivation. The study led by Dragana Rogulja used the genetics workhorse, Drosophila melanogaster, to show that increased sleep loss leads to increased levels of reactive oxygen species (ROS), otherwise known as free radicals, in the gut. ROS accumulated in the fly gut no matter how sleep was eliminated, genetically, mechanically, or with RNA silencing methods against genes known to regulate sleep. The Harvard study found similar ROS accumulation in the guts of sleep-deprived mice, showing that the damage mechanism of sleep deprivation is conserved from invertebrates through mammals. The most interesting finding was that introduction of antioxidants by any means into the gut, whether by ingestion or genetic over-expression of antioxidant enzymes, eliminated the lethality of sleep loss. With high levels of antioxidants in the gut, flies could be kept constantly awake and retain a normal lifespan.
The gut ROS study by the Rogula lab is fascinating but also raises yet more questions about the lethality of sleep loss, passing the uncertainty on to the next level of detail. Are gut ROS a byproduct of normal cellular processes which sleep acts to clear out daily (and therefore loss of sleep results in increased ROS)? Or are the gut ROS normally at a low level and somehow sleep deprivation directly causes the increase in ROS?
And while we speak often and casually about antioxidants in our diets (with my favorite new revelation being that dark chocolate is loaded with them), in our modern sleep-deprived economic society, this newly discovered ability of antioxidants to reverse the lethal effects of sleep loss may be among the more powerful reasons to add them naturally to our daily routines. Poor sleep is thought to underlie a whole host of health problems and ultimately leading to low quality of life and premature death. Of course, we ideally should wait for the field to progress and for double-blind, placebo-controlled, randomized clinical studies for confidence that antioxidants are indeed useful in humans as well in reversing the damage of sleep deprivation.
I think Maria Manasseina would have thoroughly appreciated the evolution of sleep research over the past century. While working at the Polytechnic Institute in Vienna from 1870-71, she discovered cell-free fermentation of alcohol (decades before Eduard Buchner who received the Nobel Prize for his claim to the discovery of cell-free enzymatic action driving fermentation without ever citing Manasseina’s work). Her obvious comfort with experimental and quantitative approaches to difficult questions suggests that she would have been very receptive to the blossoming of Mendellian genetics that occupied early 20th century biology soon after her death in 1903, followed by rapid advances in molecular biology spurred by the incursion of physicists into the study of life after World War II, and then the genomics revolution enabled by the contributions of computer and data scientists to the analysis of massive amounts of data produced by biology experiments. Experimental methods have clearly evolved with the advent of electrode-triggered mechanical sleep disruption, to genetic manipulation of model organisms that disable sleep pathways upon exposure to heat or light, to application of pharmaceuticals like RNAi to disrupt sleep regulatory genes, but the goal remains the same: disrupt sleep and find out what went wrong in the animal, whether puppies or rats or fish or flies.
Manasseina’s experiments were horrific and cruel, and I am sure they were extremely difficult to for her to do. Nonetheless her contributions were seminal and the field continues to make progress despite the inherent difficulties.
Here are a few more links and articles that inspired and informed my blog post, aside from the ones that are already hyperlinked within the body of my post:
Yorumlar