Ocelloids – The Eyes of the Carnivorous Algae!!
- ScienceDuuude
- Sep 12, 2020
- 10 min read
Updated: Sep 17, 2020
Photosynthetic and predatory algae with camera-like eyes are an evolutionary conundrum
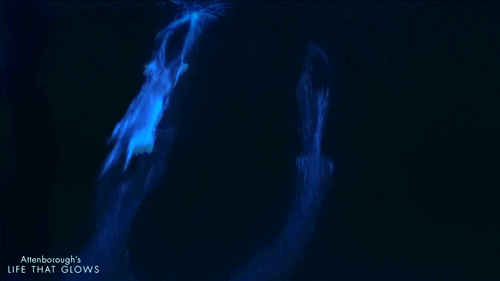
1. Peepers on plants…
Dinoflagellates are awesome. They are diverse, microscopic, single-celled algae. We know of them because some cause red tides, poisoning shellfish beds and sometimes the people who eat the tainted mollusks. They are one of the causes of ocean bioluminescence, where at night bright, fluorescent blue-green waves crash to shore and kayakers or surfers stir up glowing tracks in the water. Many dinoflagellates have spiky armor plates, and all are mobile, using whip-like cords called flagella from which they get their name. And they are photosynthetic, able to convert sunlight, water and carbon dioxide into chemical energy like sugars.
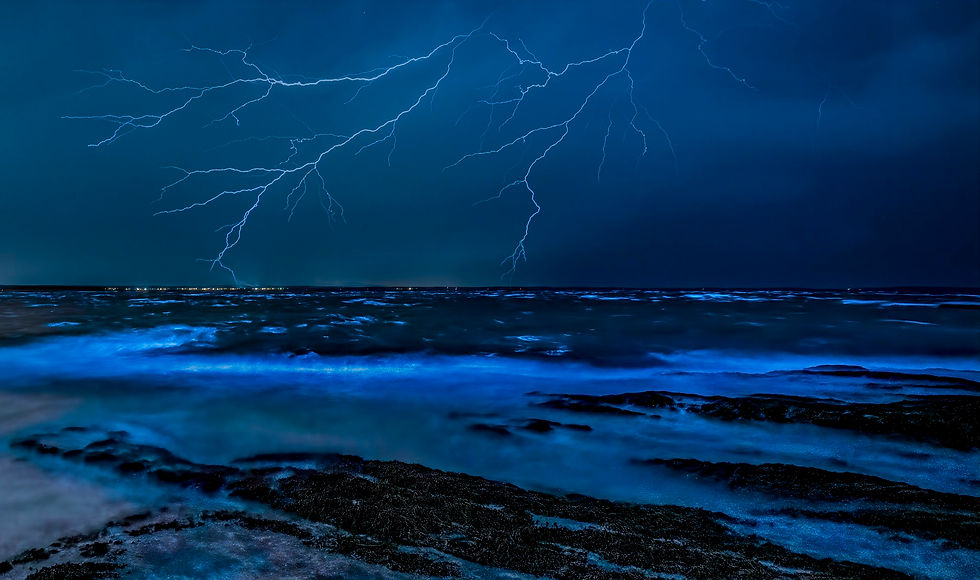
Bioluminescence glowing blue in the waves, as an electrical storm pierces the sky at Jervis Bay; NSW South Coast; Australia (Photo by Trevor McKinnon on Unsplash)
A subset of dinoflagellates is particularly awesome. They are both photosynthetic and predatory, probably consuming other dinoflagellates. This is a tiny and rare family called the Warnowiaceae, and are among the most complex single-celled organisms we know of.
Warnowiids (the name for members of the Warnowiaceae family) employ a range of complex sub-cellular machines such as:
- nematocysts – which throw out prey-capturing filaments
- trichocysts – which extrude threads in response to stimuli
- pistons – which rapidly extend and contract a long rod that may be used for locomotion or to capture prey.
But the most spectacular feature of these single-celled, mobile, predatory plants, is the ocelloid, or eye.
2. Ocelloids are like eyes…
Ocelloids are subcellular structures in Warnowiid dinoflagellates and have all the components we associate with camera-like eyes in vertebrates like us, and in cephalopods like squid and octopus:
- cornea – the clear outer layer of the eye which gathers light
- iris – rings which limit light like the diaphragm shutter of a camera
- lens – which focuses incoming light
- retina – which receives the focused light and generates a chemical image signal.

Comparison of warnowiid oscelloid (1) and the vertebrate eye (2) by Hayakawa et al., 2014.
Of course, one of the first questions we want to ask is: what do these eyes see? The answer is we don’t know. Warnowiids are rare, difficult to keep alive when captured, and so far, impossible to raise in culture. So, it is very hard to test the creatures now, to determine whether these microscopic eyes function and what the Warnowiid actually sees.
Limited tests to date suggest that these complex organelles are light-sensitive, are damaged by strong light, and contain proteins called rhodopsins that are associated with vision in all the eyes we know of. Other circumstantial data show that Warnowiids contain digested remnants of other dinoflagellates, suggesting that they are hunters and perhaps use their eyes to capture prey. Of course, we know of microscopic hunters that do not need eyes to chase their prey, so the question remains: why do Warnowiids need such complex eye-like structures?
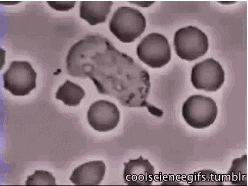
Neutrophil, a white blood cell chasing bacteria by chemical trails (coolsciencegifs)
3. How to build ocelloids…
Even more fascinating than knowing a single-celled photosynthetic alga has an eye, is how it is built. For our own eye, we have a pretty good understanding of the cells and proteins making up the different optical structures. For example, we know our lens is made of a single layer of epithelial cells on the surface and the bulk is a transparent protein called crystallin; we know the retina is made of cone and rod cells packed with light-sensitive rhodopsin proteins which detect visible-light photons.
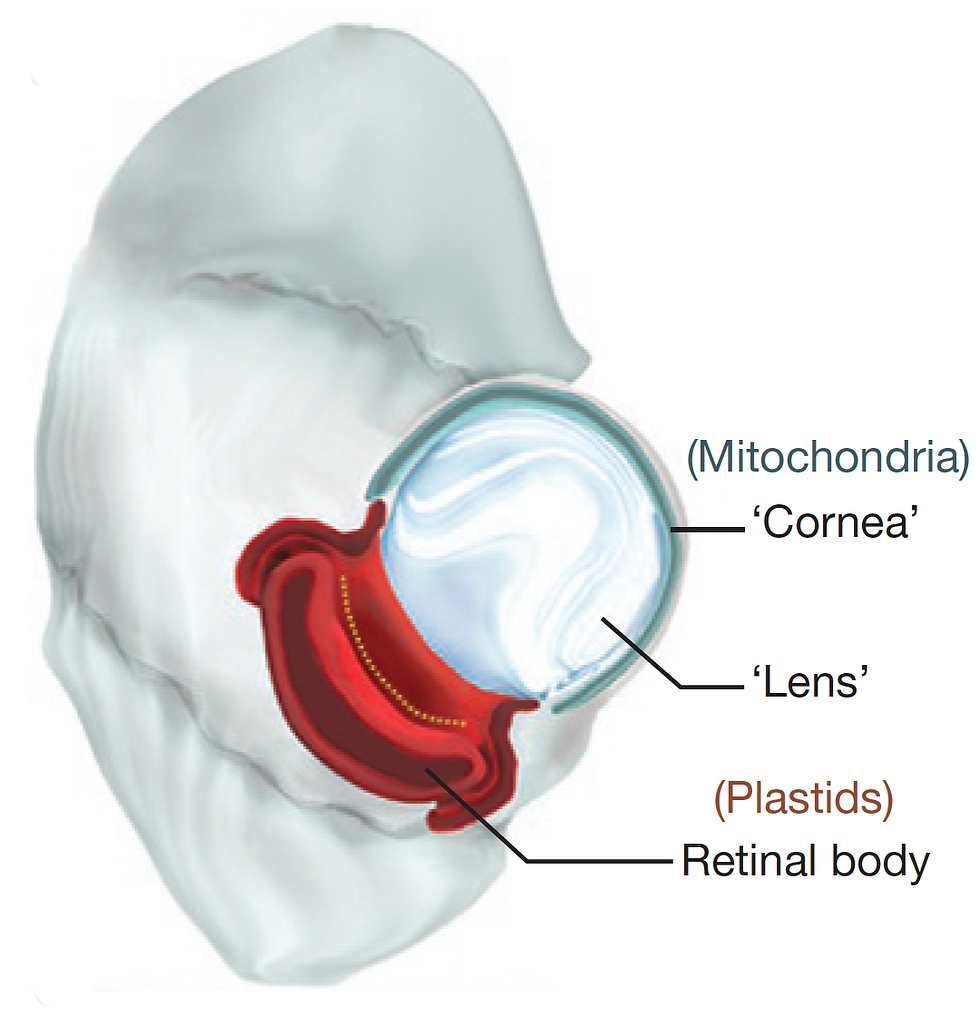
Illustration of the ocelloid eye of the dinoflagellate Nematodinium (Gavelis et al., 2015)
The sophisticated camera-like eye in Warnowiids cannot be made of cells, since the entire organism is a single cell. So how is its eye made?
Two papers, one by Hayakawa et al., published in 2014 in the journal PLOS ONE, and another by Gavelis et al. in 2015 in the journal Nature, help us understand how these ocelloids are built.
It turns out Warnowiids have repurposed several well-known sub-cellular components into the service of vision.
For example, all eukaryotic cells (cells with a nucleus) have mitochondria, the energy-producing organelle within each cell.
It turns out in the Warnowiids, some of the mitochondria are drafted into making the equivalent of the light-gathering cornea in the ocelloid.
The lens-like structure in the ocelloid is a structure called a hyalosome, and appears to be made from a common cellular structure called a vesicle – which is a membrane-bound sac.
The iris-like structure in an ocelloid is formed from something called a thylakoid. A thylakoid is a flattened pigmented sac where the light reactions of photosynthesis occur.
And the all-important retina, the structure in our eye that receives and processes light into chemical signals, has a similar structure in Warnowiids made of a photosynthetic organelle called a plastid. The photosynthesizing chloroplast in all the green plants we are familiar with, is one example of a plastid.
Of all the components in the Warnowiid cell making the ocelloid, the mitochondria and plastids are the most interesting. They are endosymbionts - once free-living bacteria-like organisms that were taken up into a host cell and passed on to their progeny.
4. Endosymbionts – you will be assimilated…
One of the most controversial and revolutionary biological concepts was the idea that major evolutionary leaps can occur by a process called endosymbiosis.
In 1967, Lynn Margulis was an adjunct assistant professor at Boston University. She had been married to Carl Sagan between 1957 to 1964, and in 1967 married physicist and crystallographer Thomas Margulis. 1967 was also the year her highly controversial paper titled “On the Origin of Mitosing Cells” was published in The Journal of Theoretical Biology, after being rejected by fifteen other scientific journals (the paper was attributed to Lynn Sagan, her name when the journal first received her paper).
But publication of her opus on endosymbiosis was just the beginning of a lonely multi-decade long campaign for her ideas to not only be accepted, but even heard.
In the 1967 paper, Margulis argued that within “higher” eukaryotic cells some critically important organelles were once free-living prokaryotes (cells without a nucleus like bacteria). These free-living cells were symbiotically engulfed by other cells to eventually become essential parts of the complex eukaryotic cell.
Most of us are familiar with the mitochondria, the power-plant of all eukaryotic cells from yeast to human. Margulis’s hypothesis said that billions of years ago, very early in the evolution of life, photosynthesis developed to capture the energy of sunlight. A byproduct of photosynthesis is oxygen which was toxic to most life at the time. Soon, photosynthesizers produced so much oxygen, they created an oxygen crisis. Aerobic (oxygen-using) prokaryotes evolved to survive in the new oxygen-laden environment. Some anaerobic (oxygen-avoiding) prokaryotes learned to survive by ingesting aerobic cells and figured out how to co-operate. The aerobic cells became our mitochondria. Eventually, this symbiotic relationship became essential to survival. The term biologists use is obligate endosymbiosis.
The acquisition of endosymbiotic mitochondria was the first step to more complex cells with a nucleus, the eukaryote.
The other classic endosymbiont Margulis wrote about are less discussed now, but as essential to life today. After the oxygen crisis, some of the newly evolved eukaryotes engulfed one or more prokaryotic photosynthesizers which became obligate endosymbionts known as plastids. One of their descendants are the chloroplasts which we find in all higher plants today.
A third example is not so critical to our story of ocelloids, but is interesting and related nonetheless. Spiral, undulating cells called spirochetes (like those today which cause syphilis and Lyme disease) were engulfed by eukaryotes and became the motor driving their mobility. That motor is now the flagella, the whip-like driver of cells like our bioluminescent dinoflagellates.
Lynn Margulis died in November, 2011 of a stroke. Her hypothesis had finally been experimentally confirmed in an important 1978 paper by R.M. Schwartz and M.O. Dayhoff. Since then her endosymbiosis theory gradually and eventually became accepted.
British biologist Richard Dawkins said this of Margulis in 1995:
“I greatly admire Lynn Margulis's sheer courage and stamina in sticking by the endosymbiosis theory, and carrying it through from being an unorthodoxy to an orthodoxy. I'm referring to the theory that the eukaryotic cell is a symbiotic union of primitive prokaryotic cells. This is one of the great achievements of twentieth-century evolutionary biology, and I greatly admire her for it.”
5. What do they see…
The presence of a complex structure like an eye in an organism does not necessarily predict a complex behavior. For example, the box jellyfish has 24 eyes of various types, including eyes which are very similar to vertebrate eyes like our own. Box jellyfish eyes include corneas, lenses and retinas. However, their behavior appears limited to simple automated responses to shadows or avoiding objects.
A 2011 paper suggested one set of lensed eyes in the box jellyfish is used for navigating. Box jellyfish prefer a narrow zone near mangroves, and when moved away to open water will rapidly move back toward the shadow of the mangrove – if it is within sight. This happens even though jellyfish lack a brain or central nervous system.
Box jellyfish do contain one of the most complex nerve nets among the jellyfishes (all jellyfish contain a sparse, radially distributed net of neurons). But let’s put box jellyfish complexity into perspective. They have four identical sensory structures called rhopalia. Each rhopalium manages six eyes (an upper and lower lens eye, two pit eyes, and two slit eyes). Each rhopalium is associated with a nerve ganglion containing 1000 neurons. So, the box jellyfish which can grow to about 8-12 inches in diameter, has a total of about 4000 neurons associated with their not-a-brain neural net. Perhaps an Einstein among jellyfish. But the tiny fruitfly, about 1/8 of an inch in length, has about 100,000 neurons in its brain.
We see that a complex camera-like eye in the box jellyfish is associated with what appears to be a severely underpowered neural machinery which may not drive a complex form of vision or corresponding behavior. Nonetheless, the sensing of light signals is clearly associated with a direct physical behavior, even if it is a simple hard-wired movement toward or away from something.
It is fun to speculate on whether a complex nerve net evolved along with a complex eye in box jellyfish to provide a very simple form of vision. And what does that vision “look” like?
For our Warnowiid dinoflagellate single-celled algae, there are no neurons, no neural nets, much less a brain. Here’s an interesting microscopy image for you. This is a 3D reconstruction of an ocelloid showing each color-coded component of the eye:
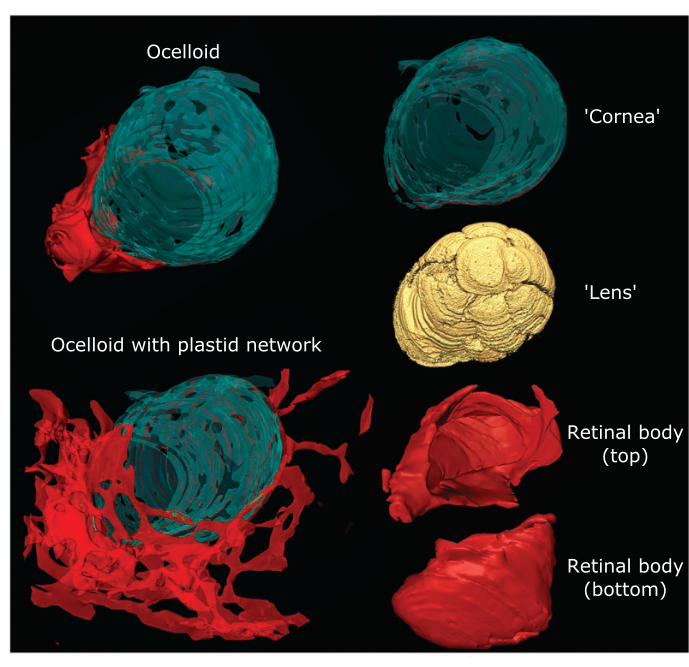
We mentioned that the equivalent of our light-sensing retina is built in the Warnowiid using the photosynthesizing endosymbiont plastid, shown in red in the lower right of the figure by Gavelis et al.
But check out in the lower left of the same figure – the plastid also shows up as an incredibly complex network that extends far beyond the retinal body. Why are these plastids forming a network? Is it possible that within the Warnowiid cell, these sub-cellular plastids are the equivalent of a neural net? Is the light signal captured by the plastid retinal body, somehow transferred to the plastid network, and processed? Do the plastids somehow convey that information to other organelles, the flagella, the piston, etc.? Does that network somehow control the hunting behavior of these already complex Warnowiids? These photosynthesizing, predatory algae with eyes?
6. Evolution and the eye…
Charles Darwin used the eye extensively in his book On the Origin of Species. He focused on our camera-like eyes in a section titled “Organs of extreme perfection and complication”, where he said:
“To suppose that the eye with all its inimitable contrivances for adjusting the focus to different distances, for admitting different amounts of light, and for the correction of spherical and chromatic aberration, could have been formed by natural selection, seems, I freely confess, absurd in the highest degree."
Biologists from Darwin up to the recent authors of the Warnowiid papers all expressed marvel and shock at the complexity of the eye. And today they wonder at how a single-celled organism can evolve a structure comparable to the complex camera-like vertebrate eye.
Something is amiss.
Consider that a camera-like eye with a cornea, lens, and retina has evolved independently in the following diverse groups of organisms:
- single-celled Warnowiid dinoflagellates
- box jellyfish
- cephalopods (squid, octopus, cuttlefish)
- vertebrates (from lamprey to fish to mammals)
Perhaps the evidence is showing us that the camera-like eye is not a particularly difficult visual structure to evolve. Maybe our eyes are not so special. They may even be primitive, basal structures, not very different from eyes in our early vertebrate ancestors, and not so highly evolved.
We don’t know the details of how the eye evolved, only that it has done so on many occasions during evolutionary time.
There are also many simpler eyes, from spot eyes, patches of pigment in single-celled organisms and many invertebrates, to compound eyes in various arthropods from crabs to insects, and more.
While the question of how the eye evolved is still an important question, perhaps a more interesting question is how the machinery to interpret the visual information evolved.
The simplest end of the spectrum are single-celled organisms which have hard-wired reflexes to various sensors such as chemical (analogous to smell/taste), mechanical (touch), vibration (hearing), and light (vision) sensors. It is safe to say these organisms do not experience senses such as smell or vision, but are merely reacting automatically to stimuli.
One question is how far in the spectrum of complexity is the strategy of hard-wired reflexes preserved? My guess is that the box jellyfish, despite the complex camera eye, responds automatically to visual signals. I believe there is no vision perception in a box jellyfish.
Humans also retain some hard-wired reflexes to senses. Touch a hot stove, and you know what I mean. Activate a fake snake wiggling in the peripheral vision of most people, and you will get what I believe is a hard-wired reflex. But clearly, humans have evolved a complex perception associated with each sense, a cognition and association that goes far beyond a hard-wired response.
This is not unique to humans, so the question is how that ability evolved, and how far back in time in our ancestral evolutionary tree did cognitive perception originate.
Finally, let’s consider again the network of plastids apparently connected to the retinal cup of the Warnowiid eye. Are we sure that this is not a sensory network? A network able to discriminate and choose among a variety of actions depending on the visual information? To perceive and to decide?
And if single plant-like cells like these dinoflagellates, can evolve such complex structures such as camera-like eyes and complex behaviors such as mobile predation, what else can life, in the richness of time, evolve?
As Charles Darwin said in the closing sentence of On the Origin of Species:
“…whilst this planet has gone circling on according to the fixed law of gravity, from so simple a beginning endless forms most beautiful and most wonderful have been, and are being evolved.”
Comentários