Aerosols: giving a virus a ride
- ScienceDuuude
- Jul 9, 2020
- 10 min read
Virus transmission – WHO’s on first…
It is now July. And yet, despite poorly-informed assurances earlier this year from our highest elected officials that the virus is vulnerable to heat and humidity, COVID-19 is spiking again with record high rates of infection in some parts of the country. We have now passed 130,000 American deaths from COVID-19. Amid this resurgence of the SARS-CoV-2 virus we are hearing of another possible route of infection: airborne virus. Sounds like the special forces of pathogens: highly trained and lethal viruses who insert themselves behind their host’s front lines by parachuting in on incredibly tiny droplets called aerosols.
What do we know about how this pandemic virus infects us? The World Health Organization (WHO) has acknowledged two primary routes of coronavirus infection so far.
First is what they call respiratory droplets which are droplets we exhale that are greater than 5 µm in diameter (that Greek letter mu means “micro” or one millionth so 5 micrometers or 5 millionths of a meter - I’ll show you exactly how small that is shortly). Droplets larger than 5 µm travel a limited distance before settling to the ground or onto whatever happens to be in its path. In this case, if a person is within a meter of someone infectious who is coughing or sneezing or even speaking, they may directly inhale those droplets and therefore be at risk of getting infected. This transmission route informs the WHO’s recommendations on masks and social distancing.
Second is indirect contact with surfaces on which exhaled droplets settle (such contaminated surfaces are called fomites). A person may become infected by touching a surface tainted by infectious droplets, and then subsequently touching their eyes, nose, or mouth – a very common and largely unconscious act. This second route informs the WHO’s recommendations on hand hygiene.
Just this past week, over 200 scientists cosigned a remarkable letter, submitted to the WHO, strongly supporting a third major route of infection: aerosol transmission.
Let’s dig in to see if we can gather some additional context and understand why these are real issues and risks to consider.
Droplets or aerosols – a sense of scale…
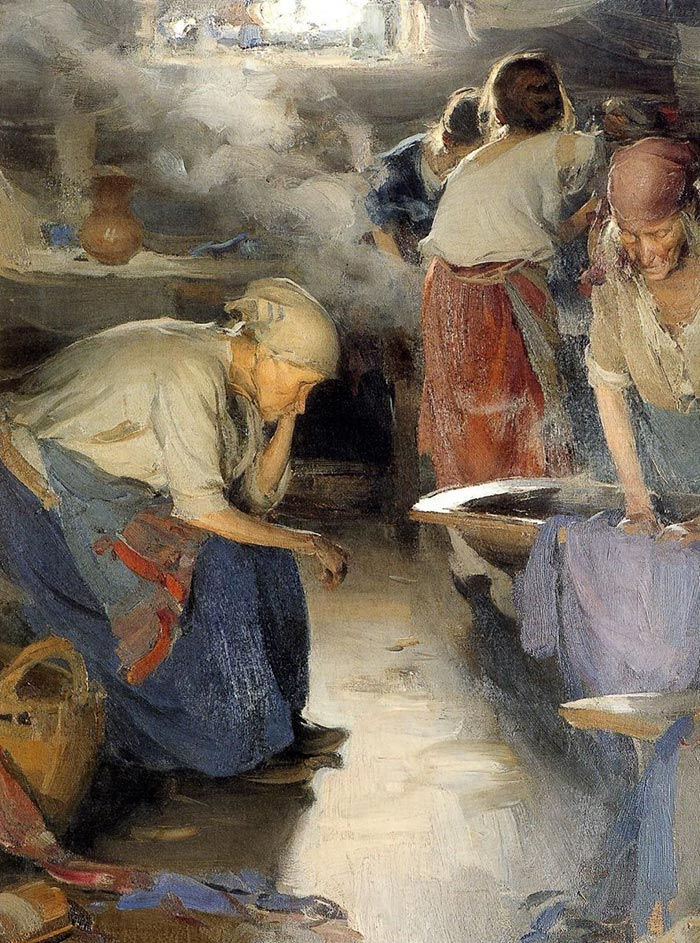
What is an aerosol?
An aerosol, in our case, is when very small liquid droplets are suspended in air. The exact definition varies and is a bit arbitrary but typically aerosols have diameters less than 1 µm. The WHO sets this limit for respiratory aerosol droplets at less than 5 µm in diameter. The important thing is that the droplets in an aerosol are so small that they remain suspended in the air and can travel relatively large distances. Larger droplets, by contrast, quickly settle out of the air by gravity, and do not travel far very – on the order of a meter or so.
Other examples of aerosols include fog or mist (aerosols of liquid water suspended in air) or smoke or dust (aerosols of solid ash, soot or other materials suspended in air). These examples provide a more intuitive idea of the distances very small aerosol particles can travel. If you smell someone smoking a cigarette from across a large room, you have a perfect example of an aerosol (in this case solid particles of soot and ash from burning tobacco) travelling far on the relatively calm air currents within a room, and entering your respiratory system through your nose.
Now imagine that the aerosol is not cigarette smoke, but mucus and saliva expelled from an infected person coughing or sneezing or even just breathing. Large droplets settle quickly not far from the person. Aerosolized spit, however, wafts across the room on the gentle currents of the air conditioner. Without the acrid smell of burnt tobacco, you have no indication that you are inhaling droplets laden with viral particles. The very small size of aerosol droplets is the key to how they move and get distributed like perfume or smoke or steam across a room, ignoring social distancing assurances.
How big is 1 µm? I said already that is one millionth of a meter. But that doesn’t really mean anything to most of us. So, let’s see if we can get a sense of scale. One way to do that is to use a series of zoomed images – let’s start with magnifying a circle 1 mm in diameter (1 mm is equal to 1000 µm).
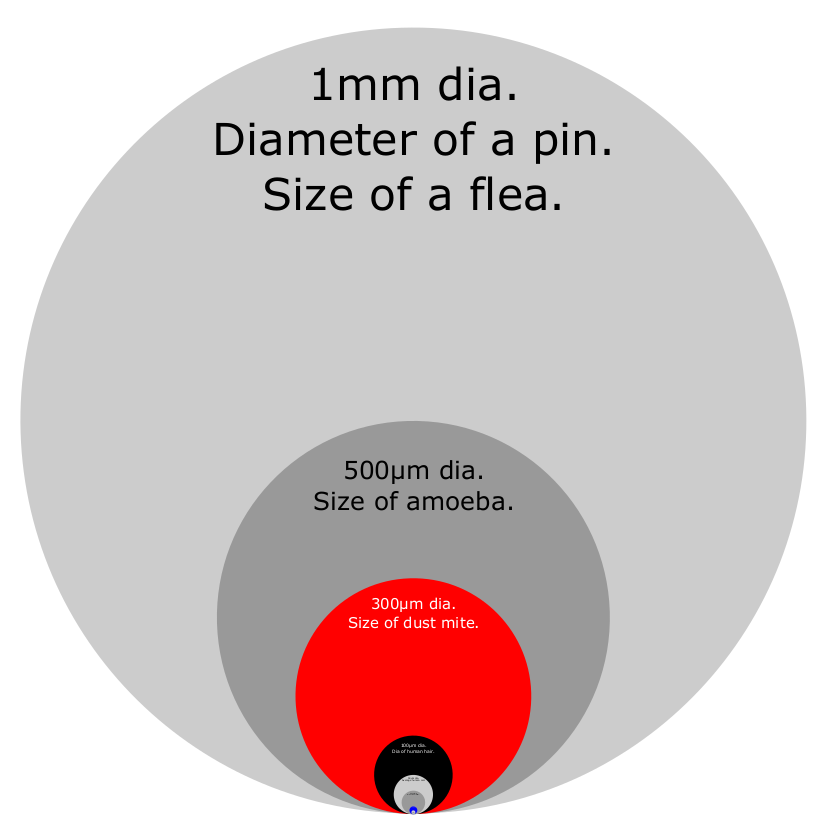
The largest light gray area represents a magnified 1 mm diameter circle which is approximately the size of a flea or the head of a pin. The circles and text are scaled equally, so the dark gray circle and the text within it is shrunk to 50% or half of the diameter of the 1 mm circle and is about the size of an amoeba. The red circle and text are 30% of the largest circle and is about the size of a dust mite. The black circle and illegible text are 10% of the largest circle, and if you could read it you would see that it is about the average size of human hair. Let’s zoom in 10X so we can see the black circle in better detail.
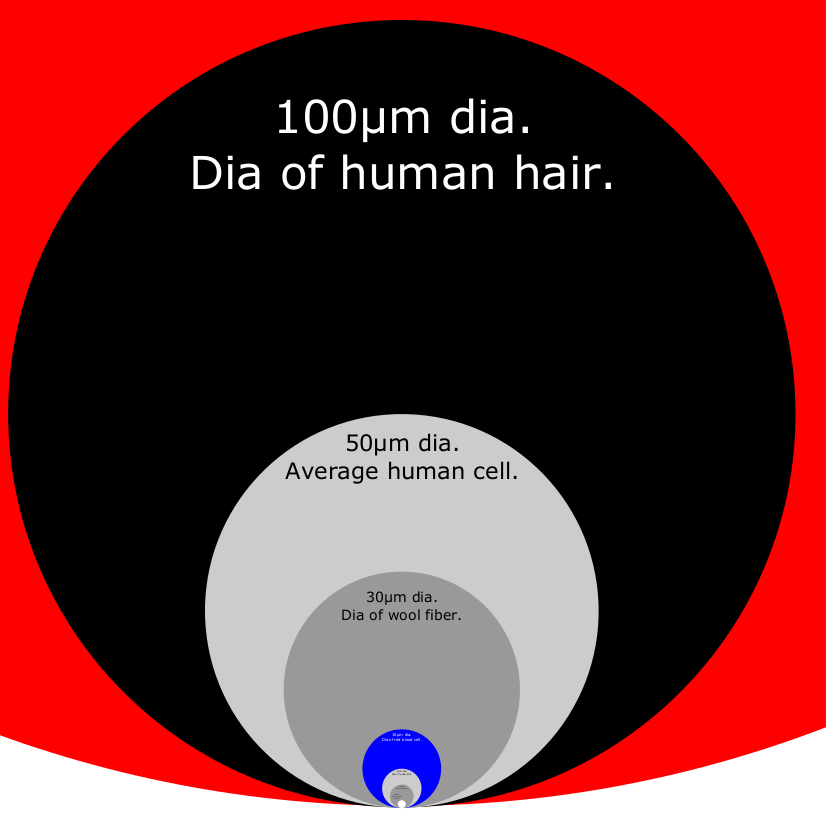
Here we have zoomed into the black circle. The red circle is mostly cropped out of the image, and relative to the average diameter of human hair, a human cell is about half its diameter, and a wool fiber is about 30%. The blue circle is 1/10th the diameter of the black circle. If we could resolve the text in the blue circle, we would read that it is about the size of a red blood cell. Now let’s zoom in another 10X to see the blue circle in detail.
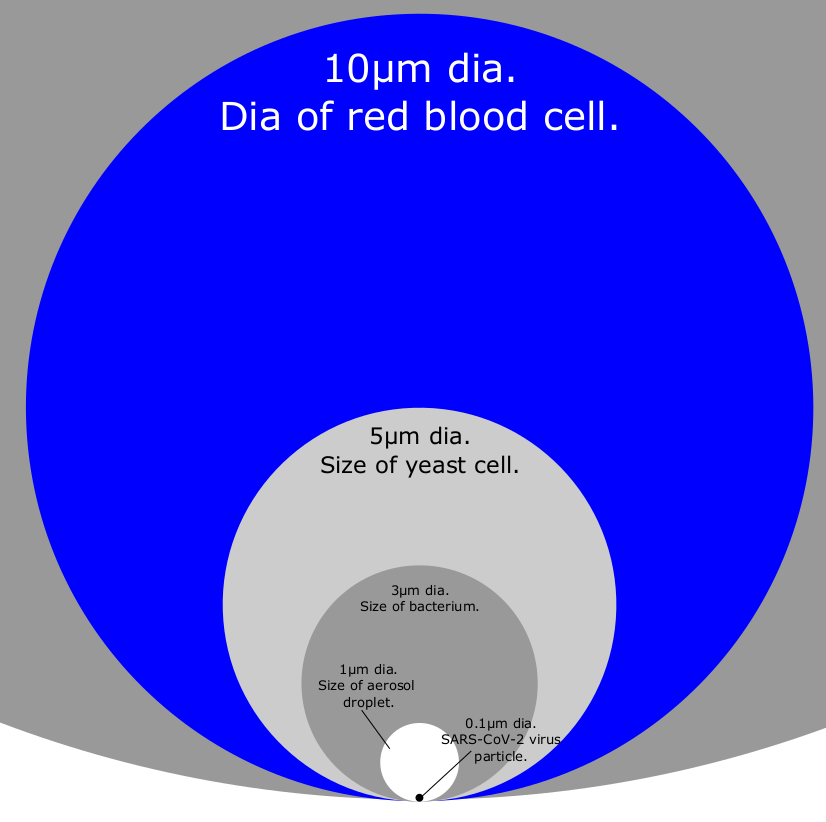
We have magnified the blue circle, which in the first image of this series was only a couple pixels across and just barely resolvable. We can see that a yeast and bacterium are about 50% and 30% respectively of the diameter of the red blood cell. And the smallest white circle is 1 µm in diameter, or 10% of a red blood cell. That white circle is the approximate size of an aerosol.
Just to complete the size scale comparisons, the SARS-CoV-2 virus causing the current pandemic is about 0.1 µm in diameter, or 1/10th the diameter of the aerosol we illustrated above. It is shown as the black dot at the very bottom of the white circle. If we zoomed into the 1 µm white circle representing the aerosol and made it as large as the blue circle, then the SARS-CoV-2 virus would be the size of the white circle.
From length to volume – a race between squares and cubes…
We have discussed only the diameter of a circle in each of the zoomed images above as a way to get a sense of the size of an aerosol droplet. A diameter is a linear dimension – a length. An aerosol is a spherical droplet, and its behavior is driven by geometry other than just diameter.
We are interested in how small spherical droplets behave, and for this we need to take a quick look at areas and volumes, and that takes us to a topic first documented by the great scientist Galileo Galilei in his book Two New Sciences, his square-cube law: as a shape grows in size, the volume grows faster than the area.
How does the area of a circle, for example, scale (increase or decrease) with diameter? Notice that the circle that is ½ the diameter of the large circle is NOT half the area – it is much less than ½ the area. Go to the last image that zoomed into the blue circle which we said was 10 µm in diameter. The light gray circle is 5 µm in diameter, or half. You can cut the large blue circle in half – draw a horizontal line tangent to the half-sized circle and you will have cut the blue circle in half – and you will see that the 5 µm circle is half the diameter of the 10 µm circle, but the area is much less than half. How much less?
If we remember our geometry, we know that the area of a circle is pi times radius squared (or half-diameter squared). So, if the diameter of one circle is half of another, then it has a quarter the area. If the diameter of a circle is one tenth of another, it has one hundredth the area. As you increase or decrease the diameter of a circle, the area changes as the diameter squared.
And – why are we talking about areas? Why is area of a droplet important for an aerosol? Remember that this droplet is moving through air. The drag force exerted by air on an object is directly related to the object’s area – imagine the difference between pulling a small parachute versus a large parachute through the air. The force of air on a droplet increases or decreases as the diameter squared.
The math behind calculating aerodynamic forces on droplets is captured in what is called the Navier-Stokes equation, essential in engineering anything from a fighter jet to an aerosol can. This fundamental piece of math was a breakthrough in fluid mechanics in the late 1800s, derived by French mechanical engineer and physicist Claude-Louis Navier and Irish mathematician and physicist Sir George Gabriel Stokes (among many other things Stokes worked with Felix Hoppe-Seyler to demonstrate that hemoglobin in blood carried oxygen, a discovery that I covered in a previous blog post here).
Now how about volume? Remember that volume of a sphere is proportional to the diameter cubed. So, if the diameter of one sphere is one tenth of another, it has one thousandth the volume (and, as we just saw, one hundredth the area).
Why is volume important? The mass of an object is proportional to the volume. And mass is what gravity acts on, so the force of gravity on a droplet increases as the cube of diameter.
And going back to Galileo’s square-cube law, as a shape grows, the volume grows faster than the area.
The force of gravity therefore increases much faster than aerodynamic forces as diameter increases. Beyond a certain size, larger droplets begin to quickly drop out of the air rather than remain suspended. Aerosols, on the other hand, are so small that their weight is much smaller than the force exerted by the air in which they remain suspended.
When those tiny airborne aerosol droplets contain infectious agents like a virus, these mathy questions of area and volume and diameter relationships, and obscure Navier-Stokes equations, suddenly take on an everyday urgency.
Can aerosol-borne viruses cause infections…
Is it possible or likely that these aerosols transmit viral infections?
This question has spurred research for quite some time, and not from a recent surge of geekiness by home-bound scientists looking to cause trouble.
Other viruses such as influenza have a long history of deadliness, unpredictability and pathogenicity. The so-called Spanish Flu caused tens of millions of deaths worldwide from 1918-19. Strains of influenza A were just recently considered one of the most feared candidates for a global pandemic before SARS-CoV-2 hit late last year. Therefore, the question of whether aerosols are a significant means of spreading the flu has been researched for a long time.
Several key points have been made about aerosol transmission of the flu, which are also applicable to our current coronavirus pandemic.
First, one of the significant factors contributing to aerosol transmission of infection is that smaller droplets find their way into the deepest recesses of the lungs, the alveoli in the lower respiratory tract. The alveoli are where gas exchange occurs (hemoglobin in red blood cells swapping oxygen for carbon dioxide) during respiration and is the preferred site of influenza infection. Larger droplets become trapped higher in the upper respiratory tract. Based on this functional analysis, various groups like the WHO use 5 µm as the threshold below which they define respiratory aerosols.
The geometry of the lungs is informative in this regard, with the main airways looking like an upside-down tree with the trachea being the single trunk splitting into two main branches leading into the left and right lungs. And like a tree, the branches (or bronchi) becomes smaller at each fork, until the final twigs (called terminal bronchioles) end in the leaves (or alveoli). The terminal bronchioles are tubes that are about 0.5 mm (500 µm) or less in diameter, supporting the alveoli which look like a tightly packed bunch of grapes. An alveolus has a diameter of about 0.2 mm (200 µm) and can increase significantly during inhalation.
The diameters of 500 µm and 200 µm of the terminal bronchioles and alveoli are similar to the larger dark gray and red circles within the 1 mm circle, and are hundreds of times larger than the aerosol particles we are discussing.
Second, coughs and sneezes and even normal breathing from one person emit large numbers of aerosols that can reach the alveolar regions of the lungs in another person.
Third, an infected individual can aerosolize a very large number of viral particles, up to 10 million per milliliter, as determined by tissue culture or mouse studies.
And finally, aerosol exposure in laboratory conditions cause infections in mouse and monkey models, as well as in human volunteers. Recent mathematical models suggest that half of influenza A transmissions in Bangkok and Hong Kong households could be attributed to aerosols rather than to large droplets or fomites.
The possibility of aerosol transmission has implications for recommended mitigation efforts, including room ventilation, or N95 respirators, and to limitations of current mitigations such as surgical masks, social distancing or hand hygiene.
Not all are convinced that aerosol transmission is a significant route for influenza infections. The WHO and others feel that aerosols cause an insignificant fraction of influenza cases. The main argument seems to be the lack of data showing clinical cases being directly caused by aerosol transmission, instead of by large droplets or fomites.
Airborne coronavirus…
Circumstantial evidence for COVID-19 infections caused by aerosol continues to mount however, with recent studies tackling this issue in a couple different ways.
A recent Nature paper detected SARS-CoV-2 viral RNA in aerosols captured in two Wuham hospitals during the COVID-19 outbreak earlier this year. Although the researchers detected viral RNA, they did not prove the virus was active and infectious. Nor did the paper prove that actual clinical infections were caused by these virus-laden aerosols.
Another recent paper in the New England Journal of Medicine showed that SARS-CoV-2 virus (which is responsible for the current pandemic) as well as the older SARS-CoV-1 remained stable and viable in aerosols for 3 hours.
An earlier paper published in Nature this past April focused on the ability of masks worn by symptomatic individuals to reduce the amount of viral RNA detected in droplets and aerosols. This paper did not specifically test SARS-CoV-2, but suggested that their data from seasonal coronavirus (common cold) and other viruses was directly applicable to controlling COVID-19.
Since the SARS-CoV-2 virus is so new to science, it is not possible to have the depth of knowledge about it that we have of other potential and known pandemic pathogens. For example, an excellent review of the aerosol transmissibility of various influenza viruses shows that specific genetic changes to the virus’s receptors, known as hemagglutinin and neuraminidase, are known to affect the ability of the virus to become transmissible by an airborne path and increase the chances of become a pandemic.
We do not have anywhere near that level of understanding about the SARS-CoV-2 virus and may not for many years. The WHO’s insistence on denying a major role for airborne transmission of COVID-19 because of a lack of direct clinical evidence, while understandable, is also short-sighted and distracting from the core risk mitigation and safety priorities of the organization. There is sufficient circumstantial evidence, and sufficient health and safety risk, to accept the premise that SARS-CoV-2 can be transmitted at high frequency by aerosols, and to implement additional mitigations accordingly. We should of course still be open to evolving research data, knowing that this information will come out over years to come, and may very well contradict whatever position we take today. The question at this moment is NOT about being right or wrong. Instead the question is, knowing what little we know now, what is the best action to protect the most people.
Comments